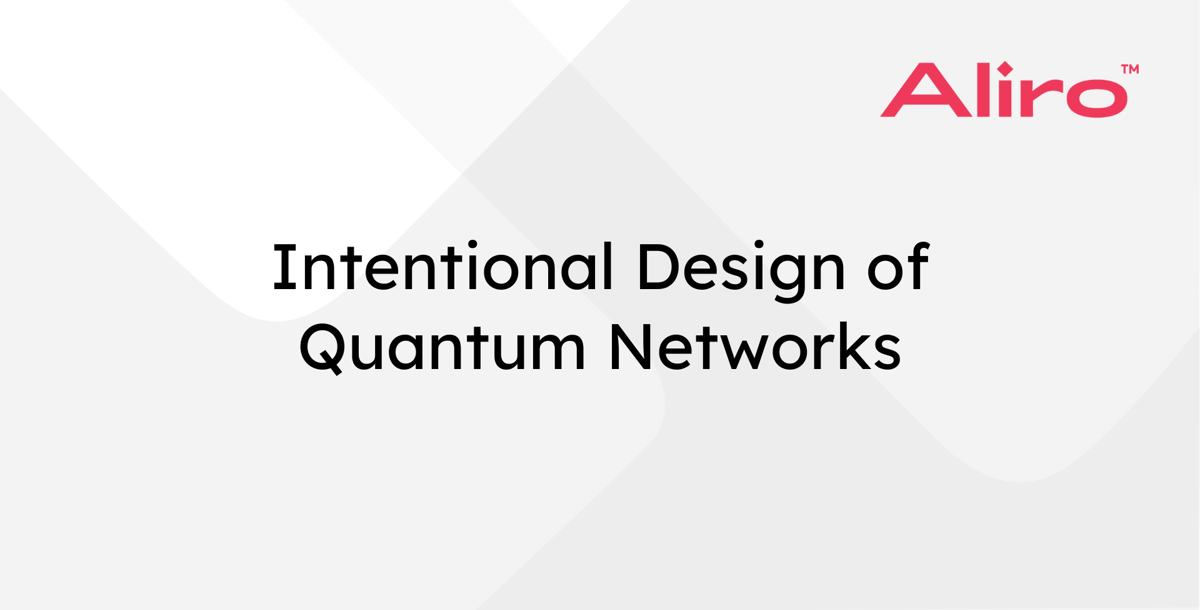
In this article Aliro Simulation Development Lead Eric Brown explains:
- The complexity you’ll encounter when designing a quantum network
- The difference between simulation and emulation in the design process
- The key decisions you’ll make during the Design and Emulation phase of building a quantum network
This article is an abbreviated excerpt from a webinar with Aliro Simulation Development Lead Eric Brown that first aired on Bright Talk. You can view the full webinar here.
The challenges of building an entanglement-based quantum network are related to the nature of the technology. These challenges come in the form of decoherence, lack of standardization, and complications that arise because of the no-cloning theorem.
Decoherence
Decoherence is a challenge because it can disrupt qubits. An example of decoherence can be seen in today's quantum memory devices. Quantum memories are used to hold onto a qubit state for use for later, but the qubit is prone to decoherence, or losing its quantum state, due to interference from the environment of the device. That interference could be related to temperature, vibrations, and other environmental factors surrounding the quantum memory. Quantum systems are very sensitive to environmental interactions, so the engineering challenge of making these devices is isolating the qubits from environmental interactions. The reason this decoherence is a problem is because it can destroy superpositions and in a sense, turn a qubit into a classical bit again.
Lack of standardization
Lack of standardization creates challenges when interconnecting hardware in a network. Because quantum technology is still nascent, there's no standardization between different types of devices and approaches. Different types of devices can have very different timescales, and therefore very fine time precision and clock synchronization between nodes is important. These devices will generally exchange quantum information in the form of light, as photons. The frequency of that light will be device specific. In order to connect different devices that act on different frequencies, it might be necessary to use transduction. In this example, quantum transduction means changing a photon of one frequency into a single photon of another frequency, while maintaining its quantum state. While challenging, this is an area that’s rapidly developing.
The no-cloning theorem.
The no-cloning theorem states that quantum information can’t be copied or cloned, at least not in the way we think of the process classically. Unlike classical information, where classical bits can be copied directly, quantum information can’t be copied without destroying the quantum information - the quantum state the qubit is in. This creates a challenge to placing nodes in a quantum network at longer distances, since signals can’t be amplified as in traditional networks. For example, if you want to send qubits (photons encoded with quantum information) to another node, they must travel through fiber optic cable or through the atmosphere. Both of these paths cause loss - photons get lost along the way. Typically, we are sending qubits in the form of single photons, so if that photon is lost along the way, the qubit doesn't arrive. Even if it does arrive, the quantum information may not arrive intact: it may have experienced noise or decoherence.
Because of these complications, it's really important to be confident a networking design is going to work before purchasing hardware and interconnecting devices. Identifying issues upfront via simulation or emulation of the proposed quantum network hardware and software will save time, money, and effort later.
High-level information to guide the quantum network design process
Before beginning an entanglement-based quantum network design, some initial information is necessary to guide the decision making process.
- Who will be using it, and what will they be using it for? For example, the network might be for internal use by an organization, or it may be designed to serve an organization’s clients. The quantum network users may want to use the network for distributed quantum sensing, quantum secure communication, for testing their own quantum device designs, or some combination of these operations. These are just a few examples of how users may wish to use the quantum network, there are many other potential applications of this type of network.
- What is the scope of the quantum network solution? This includes considerations like budget and timeline for building the network. This also includes the future scope of the network: as the technology improves and becomes more powerful, what will the next stage of your quantum network enable?
- What performance does your network actually need to have? Depending on your use cases, and how many users you want to serve, the performance requirements will change. Performance metrics include considerations like how much downtime is required, what is the maintenance cost, and the entanglement rate and entanglement fidelity that your network is distributing. Entanglement rate is the number of entangled qubits per second the network is capable of distributing. Entanglement fidelity is a measure of the quality of that entanglement. If the quantum network’s fidelity is 0.9, that means the entanglement the network generates has a 90% chance of operating successfully. Depending on your purpose, on your user applications, higher speed or quality may be desired. In general, you're going to have to make a trade off between these two metrics when you're engineering your system. If users need to have very high quality qubits to do precise experiments with, or for security where errors are unacceptable, then you’ll be prioritizing very high fidelity with your quantum network design. However, that will inherently mean a slower rate of entanglement distribution. Alternatively, if your network has lots of users that will need to be served qubits, but you can accept that maybe they're not as error-free, then a faster rate of entanglement distribution will be a priority. Understanding the trade offs between these two performance metrics in particular is important for the design process.
Designing for your specific quantum network use cases
The answers to the above questions inform the design in many important ways. Among these is how you’ll encode a qubit into a photon, and the clock synchronization requirements of your network. This topic is covered in more detail in the presentation.
Encoding Qubits
How will your network encode qubits into photons? Entanglement-based quantum networks transmit quantum information in the form of light, which means being able to encode the state of a qubit into a photonic state of light. There are many different ways to accomplish this, and they each have benefits and drawbacks. Which method you use will depend on your specific use cases and the method that aligns with your quantum networking goals. Each of the different ways of encoding qubits has different corresponding requirements for hardware, so understanding what hardware is most desirable for your use case is really key to deciding what type of encoding is ideal for your quantum network.
Clock synchronization
Quantum systems are very precise with how they interact, so quantum network nodes require a high degree of precise synchronization. For example, there are certain kinds of quantum memories that can store a qubit coming in as a photon very well, but the memory has to know when to expect that photon. Another example is in quantum repeaters performing entanglement swapping. There are various ways of achieving entanglement swapping, but it often requires synchronization down to the pico second level between when photons will arrive at a repeater. Having this high precision is a challenge, but it's doable.
Simulation vs Emulation
Once the core design decisions have been made, it's time to test how the design will work. This is where simulation and emulation can be used to determine, first of all, whether or not the design works. And, if so, then there’s an opportunity to simulate or emulate different hardware combinations and device parameters in order to optimize the performance of the design. Simulation and emulation will also give you an opportunity to experiment with control software. As a practical example, consider a quantum network design that utilizes a quantum repeater: how should the network respond if entanglement swapping is being performed but one qubit hasn't arrived when expected? Defining how the network will stop and restart that operation, how long it should wait, and the best values of any other related parameters is just as important as one’s choice of hardware. In short - a simulator or emulator should be able to accurately represent the interplay between the various hardware and software selections you’ve made based on your specific use case design decisions.
The terms simulation and emulation are sometimes used interchangeably, but there is an important difference between these two techniques. If you don't have any hardware yet, but you want to build a network, you can use a simulator to simulate how those pieces of hardware would work and interact with each other. Of course, a simulation will only be as good as your simulator. If the accuracy of the simulator isn't up to par, then the evaluation that simulation provides could be incorrect. The accuracy of a quantum networks design test improves dramatically with emulation: doing an experiment with physical hardware in combination with a simulated network. Emulation done this way will give a much more precise picture of how a physical piece of hardware would function within the context of a larger quantum network.
Use what you learn through the Design and Emulation stage to make informed decisions about the necessary trade offs in cost, performance, and node placement. Approaching each of the decisions throughout the Design and Emulation stage with intention will ensure that you create a quantum network with a data-driven-and-optimized vision at the foundations.
This article is taken from a webinar that originally aired on Bright Talks. You can find that webinar here.
Aliro, The Quantum Networking Company®, offers AliroNet™ to run entanglement-based Quantum Networks for applications such as Quantum Secure Communications (QSC), secure access to clouds and data centers, networking of quantum computers, and networking of distributed quantum sensors. AliroNet is also used to implement comprehensive Advanced Secure Networks which include Post-Quantum Cryptography (PQC). Aliro provides quantum network simulation as a professional service or as an on-premises offer. AliroNet™ users include utility companies, telecommunications providers, public sector organizations, enterprises, and researchers who are simulating, designing, piloting, orchestrating, and building the world's first quantum networks. Visit us at www.AliroTech.com.
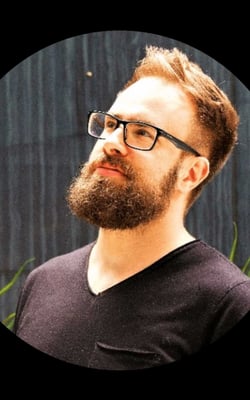